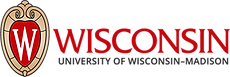
Kuang Lab
Adaptive Materials & Advanced Manufacturing
-We engineer forms and functions of soft (bio)materials for sustainability and healthcare
RESEARCH MISSION & INTEREST
Our research focuses on understanding and developing transformative manufacturing technologies for soft (bio)materials and systems, aiming to solve societal challenges in sustainability and health. Through experimental, theoretical analysis, and computational tools, we study the functional responses of (bio)polymeric systems to external stimuli (such as heat, light, chemical, magnetic, mechanical, and acoustic fields) and establish high-quality synthesis process-structure-property relationships. The objective is to guide the design of multifunctional composite (bio)materials and innovate advanced manufacturing technologies. Research directions include stimuli-adaptive (bio)polymeric materials, sustainable and digital manufacturing (specifically ultrasound-based techniques), and multifunctional devices and (bio)systems. Applications extend to aerospace, soft robots, biomedical devices, drug delivery, and tissue engineering.
Understanding the stimuli-polymer interactions and establishing process-structure-property linkage by advanced characterizations and modeling tools for accelerating the design of dynamic (bio)polymers and composites with multifunctionality;
Converging adaptive (bio)polymers, operando characterizations, and adaptive control to develop sustainable and digital manufacturing, particularly ultrasound-based;
Multifunctional devices and (bio)systems
Leveraging adaptive materials and advanced (de)manufacturing to create structures and systems with biomimetic multifunctionalities, such as self-healing/welding, reshaping, degradation/recycling, actuation, growth, and cell-instruction, for aerospace, soft robots, biomedical devices, drug delivery, and tissue repair.

RESEARCH OVERVIEW
By 2050, the world’s population is projected to reach 9.8 billion, with the number of individuals aged 60 years and older doubling to 2.1 billion. To meet the societal demands for production, living, and healthcare, a tremendous amount of materials, including metals, ceramics, and polymers, have been widely used. (Bio)polymers and composites, characterized by their lightweight nature, ease of synthesis/fabrication, and versatile multifunctionality, tissue-matching physicochemical properties have become the preferred choice in numerous cutting-edge technologies such as aerospace, flexible (bio)electronics, medical devices, drug delivery systems, and tissue engineering. However, conventional polymer composites face substantial challenges, including limitations in multifunctional applications due to their simplistic geometries and inert properties, as well as the environmental concern arising from the extensive use of plastics-based products and devices, which are estimated to reach approximately 590 million metric tons by 2050, with only 14% being recycled.
Natural materials and systems have the synergistic beauty of energy/material efficiency in life-cycle, superior physical properties, and intriguing functionalities for service. These materials owe their remarkable properties to multiscale heterogeneity, encompassing weak/strong bonds, soft/hard building blocks, and intricate geometries/multimaterial compositions. Inspired by the integrated form-function relationship observed in natural materials/systems, advanced manufacturing techniques, such as 3D printing, have been extensively investigated for producing engineered functional materials. These multifunctional materials, characterized by heterogeneous structures and adaptive functions (self-healing, degradation, growth, and responsiveness), hold promise in addressing the above challenges. A fundamental understanding of multiscale stimuli-material interactions is pivotal in guiding the molecular design and advanced manufacturing of functional materials and devices. Among various stimuli, ultrasound emerges as an up-and-coming option due to its ability to penetrate deep regions (up to 20 cm) with precision focusing akin to light (up to 0.2 mm). In addition to its well-known capability in non-invasive imaging/detection, ultrasound can convert into various energy forms (such as heat, light, and electricity) to drive diverse chemical, physical, and biological processes. Leveraging stimuli-responsive polymers, advanced manufacturing methodologies, ultrasound technology, and state-of-the-art modeling/machine-learning techniques, our research aims to propel sustainable and digital manufacturing of multifunctional soft materials/systems. This endeavor targets advancing material/energy sustainability and enhancing precision healthcare with higher safety, accuracy, and efficacy in medical applications.